Hello all! I decided that it was about time to break into the World of Blog! I have a number of topics in mind for the future, which will focus on primarily on paleontology, geology, biology, zoology, etc. Many of you know very well that I tend towards the vertebrate paleontology side of things, however; I do love me some invertebrates! For those of you who weren’t aware, the username “Isotelus” is actually a genus of Asaphid trilobite, the main inspiration being Isotelus rex, the largest trilobite species currently known (My profile picture is as it is because the bird amuses me). In light of this astonishing revelation, my very first post will be a response to the myriad of creationist websites I have come across that seek to disprove evolution using trilobites as an example. As such, this post is not a direct response to any one particular article I’ve read, but a general statement on their overall position.
Trilobites represent one of the earliest arthropod groups in the fossil record, appearing in the lower Cambrian and persisting until the Permian-Triassic extinction event (520 MYA – 252 MYA). Comprising of roughly 20,000 species and 5000 genera, they were among the most successful group of arthropods to date. While an overall highly complex and sophisticated animal, a number of features identify trilobites as a basal member in relation to living relatives. Despite the often spectacular levels of preservation of trilobites (due primarily to their hard exoskeletons), the fact of their extinction can impose great difficulty in attempting to examine and interpret components of the body in relation to function or behaviour, especially when it involves microscopic structures, such as lenses of the eye or layers of the exoskeleton. Nevertheless, the trilobite eye, body plan and associated structures, as well as certain physiological processes including moulting and development, can be successfully compared with those of modern arthropods, which functions to demonstrate the set of primitive and derived characteristics that set the groups apart. Insects, crustaceans, and xiphosurans (horseshoe crabs) will be included here primarily, as most evaluations center on these groups in particular. This brief assessment also serves as a response to certain pseudoscience proponents who falsely claim the complexity of trilobites and modern arthropods is evidence against evolutionary processes. Advocates of creationism and intelligent design tend to focus only on the eyes of trilobite and neglect the rest of the animal, justifying the need to cover other aspects of the body and exoskeleton.
Eye Structure and Function
From personal experiences/encounters, the trilobite eye in particular is sometimes used by creationists to claim evolution has not occurred, primarily because they and modern representatives have functionally the same complex, perfected visual design. According to this view, trilobites were not primitive creatures, keeping in mind that “primitive” here is defined incorrectly. Taxonomically speaking, primitive or basal indicates a character that is closer to the ancestral condition, and relative degrees of complexity or superiority do not apply. In this context, the highly developed trilobite eyes were not necessarily inferior in their visual capacities relative to modern derived forms; however, there are many aspects of the overall structure that are nevertheless primitive in comparison to crown arthropods.
The majority of all arthropods have compound eyes that are comprised of a series of optical units called ommatidia, which themselves contain a variety of structures and function overall as photoreceptors. Trilobites are no exception, and are some of the earliest animals in the fossil record to show a more complicated version of the visual complex. While varying wildly in terms of general shape and placement, three types of eye are present in trilobites (see this site for a more detailed introduction to trilobite eyes, as well as some great pictures http://www.trilobites.info/eyes.htm). Holochroal eyes were the simplest form and by far the most prevalent type characterized by numerous closely packed lenses with a single outer corneal surface. This type is considered to be basal to the trilobite group and was likely associated with a benthic lifestyle (Clarkson et al., 2005). Schizochroal eyes occur only in one suborder and are distinguished from the holochroal type by a fewer number of lenses separated by sclera and each with an individual corneal surface. Both schizochroal and abathochroal eyes were derived from a holochroal ancestor and likely resulted from paedomorphosis (http://en.wikipedia.org/wiki/Neoteny), as fossils of juvenile holochroal species have been found with schizochroal and abathochroal eye types (The abathochroal eye appears only in a certain group for a short time, and so will not be dealt with here)(Thomas, 2005). Trilobite eyes are typically compared to those of horseshoe crabs, which retain a number of primitive traits, including potentially the most basic eye characteristics relative to other extant arthropods (Schoenemann and Clarkson, 2013). This comparison is not always well-supported, as schizochroal eyes are so unique that optical theories typical of many modern taxa cannot always be successfully applied (Fordyce and Cronan, 1993). However, detailed preservation of microscopic structures in a number of trilobite fossils has since reinstated xiphosurans as a useful structural analogue (Schoenemann and Clarkson, 2013). In terms of structural composition, the lenses of trilobite eyes were uniquely made of a single immobile calcite crystal formed in a biconvex shape to counteract aberration, rather than a proteinaceous crystalline cone typical of all insects and most crustacean groups (Nilsson and Kelber 2007). Additionally, trilobite ommatidia likely connected to a single photoreceptor, indicating both holochroal and schizochroal species had simple apposition eyes, which is considered primitive for Athropoda, and present in Limulus (horseshoe crab) and some members of other groups (Fordyce and Cronan, 1993; Nilsson and Kelber 2007; Schoenemann and Clarkson, 2013). Both trilobites and Limulus ommatidia also potentially show a star-shaped arrangement of tubular, light-collecting rhabdomeres associated with a modified sensory receptor (eccentric cell). The above similarities, as well as the similar size and number of ommatidial elements, suggest Limulus has inherited and maintained a comparatively primitive visual system (Schoenemann and Clarkson, 2013). The structure of the trilobite eye, while complex and highly functional, is clearly not as advanced as most modern arthropods.
Schizochroal eye typical of phacopid trilobites, from http://www.trilobites.info/eyes.htm

Body Plan
Trilobites were so named because of the three lobes consisting of the cephalon (head), thorax, and pygidium (tail) (see image below) (Hughes, 2003). This basic pattern of segmentation is also reflected transversely in the axial and pleural lobes of the main body. This particular configuration is highly conserved across the group, with high levels of variation occurring typically in ornamentation (e.g. spines), and relative size, number, and shape of the segments and associated structures, such as the mouthparts. Unlike the majority of arthropods today, such as crustaceans and insects that occupy a great diversity of habitats, trilobites were restricted to a marine setting and evidently never invaded fresh water or terrestrial environments. This constraint is reflected in the comparatively static trilobite bauplan, which never diverged greatly from the basic roughly ovoid shape or formed highly complex structures such as wings, or showed the higher degree of morphological divergence achieved by comparable aquatic crustaceans (lobsters, crabs, ostracods, barnacles, etc). The latter may seem counter-intuitive considering how speciose and widespread the trilobites were as a whole. One potential interpretation considers their success over a long time period in correlation with their conserved and relatively simplistic body plan, which evidentially allowed them to function perfectly well in a range of marine environments, such that any significant changes or divergences were not favoured by natural selection. Evidence from trace fossils, taphonomy, and general morphology suggests the majority of trilobite species were likely feeding on particulate matter in a seafloor environment, which may at least partially explain both the relatively unchanging body plan and holochroal eye morphology (Hughes, 2003; Clarkson et al., 2005).
Soft-tissue preservation and ventral morphology of Triarthrus, from http://www.fossilmuseum.net/trilobites/ptychopariida/Triarthrus/Triarthrus.htm;
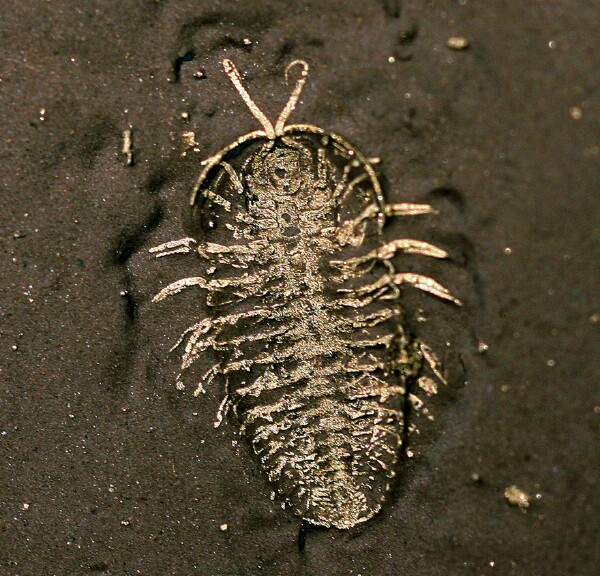
In addition to the body segments themselves, trilobite legs were biramous, consisting of two separate branches on a single limb (Hughes, 2003). Only the antennae were uniramous, and this trait along with biramous appendages seems to be the most basal arrangement among arthropods according to studies in homology and development (Boxshall, 2004).The same arrangement is also typical of crustaceans, while insect limbs are uniramous and lack a second branch. However, unlike both insects and crustaceans, the limbs of trilobites occur in identical pairs that repeated sequentially on the head, tail, and each thoracic segment, with divergence in form appearing in the uniramous antennae, or between species as a result of diet. Morphologically diverse and modified appendages typical of many modern arthropods, such as those for prey capture and consumption, reproduction, swimming, etc., are entirely absent in all known trilobite species; even those with soft tissue preservation. Although it may correlate with the relatively inflexible trilobite bauplan in association with a benthic, particulate-feeding lifestyle as discussed previously, this explanation may not necessarily account for the invariable limb morphology across all trilobite groups, including those that are interpreted as having been planktic, pelagic, or burrowing, based on other specific aspects of their morphology (such as eye size and placement) (Hughes, 2003).
Exoskeletal Structure and Development
The trilobite exoskeleton was highly mineralized in comparison to modern arthropods, which tend to rely more on organic secretions (Miller and Clarkson, 1980). In addition, the calcitic exoskeleton was fairly typical of an arthropod cuticle, with structural similarities occurring variably with modern arthropods, but was composed of only two simple, distinct main layers, the exocuticle and endocuticle (Mutvei, 1981). This same study found that a lack of a thinning endocuticle and the pores and cavities near ducts used to transport/dissolve old calcitic and organic material for reuse indicated trilobites could not reabsorb their molted cuticle. Miller and Clarkson (1980) also showed that the calcium carbonate contained in the cuticle is shed in its entirety during ecdysis. In contrast, absorption of the old cuticle in modern arthropods potentially aids in speeding the hardening process after molting, and some groups will also consume the old cuticle for nutrients and/or later incorporation. Due to the high degree of mineralization of their cuticle, trilobites were not likely able to eat their exoskeleton given the strength of their mouthparts (Brandt, 2008). The inability of trilobites to reabsorb their exuviae (shed exoskeletons) potentially left them at a particular disadvantage during moulting, as regrowth of new calcium carbonate requires a substantial amount of energy and incurs a high metabolic cost. The soft stage directly following molting may have been protracted, making them particularly vulnerable to injury and/or predation directly following their moult.
Fossil evidence shows that trilobite development is hemimetabolous, with gradual change occurring over three stages from the egg, instar, and adult phases (Hughes, 2003; Thomas, 2005). This particular form of ontogeny is primitive among arthropods, with many modern insects being homometabolous. Insects and crustaceans tend to undergo metamorphosis and grow through a series of very distinct stages. Trilobite developmental patterns in contrast are less complex in that they grow by gradually adding thoracic and tail segments with each successive molt until they reach a certain point nearing adulthood at which they continue only to increase in size. The modification across instar stages was also comparatively slight and referred to as hemianamorphic, which is seen currently in primitive crustaceans and fossil xiphosurans, and as a result is considered to be a primitive trait (Hughes et al., 2006). This process, like the eyes and body segments, also remained unchanged over the length of trilobite evolution, possibly due to compromises of selection pressures acting on other aspects of the body (That’s the best explanation I’ve found thus far, as few studies tackle this particular question) (Brandt, 2006).
Concluding Remarks
From a scientific standpoint, comparisons between fossil and modern taxa serve as a means to potentially shed light on the functional significance of certain structures and morphology across various groups. This approach has an alternate function in that it confronts factual misinterpretations of creationists seeking to find faults in the theory of evolution by claiming it evidently did not occur in perfectly-designed fossil trilobites. Trilobite eyes were well-developed and similar in many aspects to modern arthropods, and they shared a number of archaic characteristics with modern horseshoe crabs, which are generally considered to be among the most basal extant arthropods. Conversely, the trilobite bauplan, exoskeleton, and limbs were comparatively basic in contrast to morphologically diverse crustaceans and insects, and remained relatively static over time, possibly due to habitat and lifestyle. Growth and development also seems to follow a primitive pattern both in types of stages and relative degrees of change. Trilobites evidently represent a highly successful group of primitive arthropods that maintained a basic platform over time relative to modern crown taxa.
End :). I highly recommend taking a look at this website: http://www.trilobites.info/, for everything trilobite. I also apologize if the eye section of this post is a tad confusing; it’s a rather complicated topic that deserves its own blog, which I plan on addressing in the future.
Also, special thanks to Prolescum for allowing me to write this blog in the first place, and he_who_is_nobody for helping with editing.
References
Boxshall, G. A. 2004. The evolution of arthropod limbs. Biological Reviews. 79 (2): 243-300.
Brandt, D. S. 2002. Ecdysial efficiency and evolutionary efficacy among marine arthropods. Alcheringa. 26 (): 399-421.
Fordyce, D., and Cronin, T. 1993. Trilobite Vision: A Comparison of Schizochroal and Holochroal Eyes with the Compound Eyes of Modern Arthropods. Paleobiology. 19 (3): 288-303.
Hughes, N.C. 2003. Trilobite tagmosis and body patterning from morphological and developmental perspectives. Integrative and Comparative Biology. 43 (1): 185–206.
Nilsson, D.E, Kelber, A. 2007. A functional analysis of compound eye evolution. Arthropod Structure & Development. 36 (4): 373-385.
Miller, J., and Clarkson, E.N.K. 1980. The Post-Ecdysial Development of the Cuticle and the Eye of the Devonian Trilobite Phacops rana milleri Stewart 1927. Philosophical Transactions of the Royal Society of London. Series B, Biological Sciences. 288 (1030): 461-480.
Mutvei, H. 1981. Exoskeletal structure in the Ordovician trilobite Flexicalymene. Lethaia 14 (3): 225-234.
Schoenemann B., Clarkson E.N. 2012. Discovery of some 400 million year-old sensory structures in the compound eyes of trilobites. Scientific Reports. 3 (1429).
Thomas, A.T. 2005. Developmental palaeobiology of trilobite eyes and its evolutionary significance. Earth-Science Reviews. 71, (1–2): 77-93.